Heart-on-a-chip technology marks a notable advancement in the realm of tissue engineering and microfluidics. These miniature devices replicate the complex physiological responses of human heart tissues and are a subset of the broader organ-on-a-chip field. Designed to emulate the functions and mechanical properties of the actual human heart, this technology integrates living cardiac cells within micro-engineered environments, enabling real-time monitoring and analysis.
With advances in stem cell science and 3D printing, scientists have been able to cultivate cardiac tissues that beat rhythmically, similar to a human heart. Utilizing microfluidics, heart-on-chip models can simulate blood flow and the tissue’s mechanical environment, providing a dynamic system to study cardiovascular diseases, screen drugs, and possibly predict individual patient responses to therapeutics. This innovation serves as a bridge between traditional cell culture and animal testing, offering a more accurate and ethical approach to understanding the human heart and developing treatments.
Key Takeaways
- This is a groundbreaking tool in biomedical research that mimics the function of the human heart.
- This technology provides a platform for medication testing and disease modeling, reducing reliance on animal models.
- Ongoing development could revolutionize the way we approach treatment for cardiovascular conditions.
Fundamentals of Heart-on-Chip Technology
As you delve into the world of heart-on-chip technology, understanding its foundational elements is crucial. This includes not only the aspects of materials and design, but also the intricate integration of sensors and microchannels that allow for precise monitoring and functioning of the bioengineered tissue.
Design and Material Considerations
When designing a chip, you must prioritize the selection of materials that offer biocompatibility and flexibility. Two prominent materials in this regard are silicon and polydimethylsiloxane (PDMS). Silicon is valued for its structural integrity and compatibility with existing fabrication technologies. PDMS, on the other hand, is widely used due to its transparency, elasticity, and biocompatibility which enables it to host living cells and support their function.
The design of material typically encompasses microchambers that mimic the cellular environment of a human heart. Your aim here is to create a system that replicates the mechanical and biological cues of heart tissues, which involves understanding the spatial arrangement and dimensions that affect cell behavior and tissue function.
Integration of Sensors and Microchannels
Integrating the sensors is a delicate yet vital process. These sensors are used to measure electrical activity, pH levels, oxygen content, and other critical parameters that reflect the functionality of the cardiac tissue being simulated. Your choice of sensor materials and placement should be conducted with careful attention to avoid any interference with the living cells.
Microchannels within the chip are the conduits that guide nutrient flow, waste removal, and mechanical forces to the microchambers. When creating your microfluidic chip, you need to ensure that the dimensions and properties of these channels support laminar flow, which is essential for simulating blood circulation and shear stress akin to human vasculature.
Applications in Disease Modeling and Drug Development
Heart-on-a-Chip technology has revolutionized the landscape of cardiovascular research and pharmaceutical testing by providing advanced platforms for disease modeling and drug development. This includes the study and treatment of heart diseases, enhancement of drug safety assessment protocols, and the shift towards personalized medicine.
Cardiac Disease Research
The understanding of heart diseases can be significantly broadened through these systems that replicate human cardiac physiology. These systems allow detailed investigation into the complexities of cardiovascular diseases, including the nuances of contractility and vascular abnormalities. Utilizing cardiomyocytes derived from induced pluripotent stem cells, you can model different types of heart diseases, enabling closer examination of disease progression and potential therapeutic interventions.
Drug Screening and Toxicity
As a replacement for conventional animal models, this technology offers a sophisticated screening platform that aids in drug discovery and drug development. The miniaturized device provides a highly controlled environment to assess drug-induced cardiotoxicity and other forms of toxicity, thereby enhancing the predictability of drug safety before clinical trials. You can evaluate the efficiency and side effects of new pharmaceuticals, reducing the reliance on animal testing and improving drug screening processes.
Advancements in Personalized Medicine
This ground breaking tech is paving the way for personalized medicine in treating cardiovascular and vascular diseases. By constructing disease models from personalized induced pluripotent stem cells, therapies can be tailored specifically to genetic makeup, increasing the efficacy of drug treatments. This approach ensures that the patient receives medication that is most compatible with their individual heart disease profile, which could result in improved patient outcomes and a reduction in adverse drug reactions.
Challenges and Future Directions
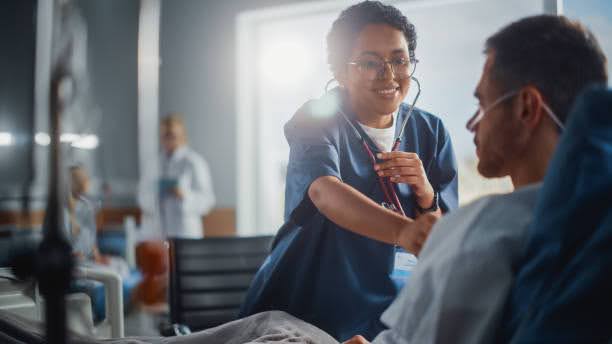
Hospital Ward: Friendly Nurse Uses Stethoscope to Listen to Heart, after a Heart-on-a-chip surgery.
Advancements in this technology confronts both technical and ethical hurdles. Your understanding of these challenges and the anticipated developments can inform your approach to their application in biofabrication and drug testing.
Heart-on-a-Chip Technical Limitations
To enhance maturation and functionality, 3D bioprinting techniques are instrumental. Yet, the resolution and consistency needed to mimic the intricate cardiac tissue structure represent significant technical limitations. You must ensure that the maturation of cardiomyocytes in vitro replicates the physical and biochemical properties of a natural heart. It’s crucial to translate the in vitro culture advances into robust biofabricated heart models that can reliably be used for drug testing and disease modeling, reducing the dependency on animal testing.
Ethical and Regulatory Considerations
The transition from animal testing to human-centric models involves navigating ethical and regulatory considerations. You should be aware that strict regulations govern human cell-derived in vitro systems. They require demonstrable benefits over traditional animal models not only in terms of predictability but also ethical acceptability. The promise of these systems lies in their potential to reduce animal use, yet their application must be carefully examined within the framework of current ethical standards.
Understanding Cardiovascular System Mechanics
In the field of biomedical research, this technology is rapidly advancing our understanding of the intricacies within the cardiovascular system. This innovative technology enables a detailed examination of the interactions among various components of the heart in both normal and diseased conditions. Cellscale has been achieving cutting-edge results through their breakthroughs in biomaterials innovation.
Recreating Cardiac Tissue Dynamics
These chips are engineered to replicate the microenvironment of your heart’s tissue, allowing the study of cardiomyocytes (CMs) and cardiac fibroblasts. By emulating the cardiac tissue, researchers can observe the response of these cells to electrical stimulation. This close examination reveals insights into the complexities of heart muscle contractions, paving the way for a deeper understanding of conditions like coronary heart disease.
Studying Blood Flow and Vascular Functions
Advanced models integrate not just cardiac cells but also endothelial cells to mimic the vasculature. This aspect is crucial for the study of blood pressure dynamics and vascular functions, which are central to conditions like hypertension. By simulating the endothelium, you gain precise insights into how the cardiovascular system responds to stressors, therapeutics, and the progression of diseases. This knowledge is critical in developing treatments for a range of cardiovascular diseases by observing how the engineered constructs react in real-time to simulated conditions.
This tech is a game-changer in biomedical innovation, transforming drug testing, disease modeling, and personalized medicine in cardiovascular research. These tiny devices, driven by stem cell science and microfluidics, faithfully mimic human heart physiology. Enabling real-time monitoring and analysis, they help researchers understand cardiac function complexities, disease mechanisms, and potential treatments with precision. The evolution of this tech promises to advance cardiovascular understanding and improve treatments globally. Cellscale has helped lead the way in characterizing biomaterials’ mechanical properties, driving advancements in medical research.